“The Covid 19 Taste” Painting (Schade, 2020)
Human beings are provided with a variety of senses that help them navigate the world around them, including five basic ones: sight, hearing, touch, smell, and taste. The organs associated with each of the latter report on certain sensations to the brain, which then translates them into understandable information, via a complex, yet fascinating process. Bright colours, a loud thud, an acute pain, a familiar flavour on the tongue or a sweet smell that tickles the nose – all these stimuli are put together into one big picture for us to identify our surroundings. However, while these systems are remarkably sophisticated ones in humans, some animals have super sensors. Felines are well-known for their nocturnal vision, elephants have the most powerful nose of the animal world, while bats rely on sound waves to hunt. Magnetoreception – the ability to detect the Earth’s magnetic field, is even considered a sixth sense that birds, along with certain mammals, reptiles, and fish, are gifted with.
The Physics of the Senses 101 series offers to explore the physical processes that make up each sense, including the so-called sixth sense, and to explain the extent to which they grant certain species ‘super-capacities’.
1. Physics of the Senses 101: Visual Perception or Sight
2. Physics of the Senses 101: Auditory Perception or Hearing
3. Physics of the Senses 101: Haptic Perception or Touch
4. Physics of the Senses 101: Olfaction or Smell
5. Physics of the Senses 101: Gustatory Perception or Taste
6. Physics of the Senses 101: Magnetoreception or a Sense without a Receptor
Experimental psychologist from Oxford, Charles Spence, explained in his review that ‘it is frequently asserted that somewhere between 75% and 95% of what we commonly think of as taste actually comes from the sense of smell’ (2015, p.1). However, he states that the empirical evidence in support of olfaction’s dominant contribution to many dimensions of taste is rarely cited when making such a ‘precise-sounding quantitative claim’ (Spence, 2015, p.1), while it can influence modernist chefs, culinary artists and designers in their approach. In fact, taste is a conflation of two complex biological phenomena: gustation – ‘the chemical sense that is mediated by specific receptors of gustatory cells within taste buds’ (Hara, 2006, p.1), and flavour perception, which involves an interaction of multiple, if not all senses: ‘[t]he sights, sounds, and smells of food prepare the body for the next meal. Hormone levels rise, stomach rumbles, and saliva starts to flow before a bite of food is taken.’ (Gravina et al., 2013, p.217)
While the experience of eating food is a highly pleasurable sensory experience, gustation remains a fundamental process for the survival of individuals for it mediates feeding (Hara, 2006). It is therefore one of the ‘most critical control systems able to regulate substance intake, evaluating the healthiness and nutritional content of food and preventing the ingestion of harmful or toxic elements’ (Pallante et al., 2021). However, recent research suggests that taste plays a deeper role than merely quality check: it is also an emotional and psychological sense (Zhou & Tse, 2020) (Chinnakkaruppan et al., 2014) (Gravina et al., 2013). This article will therefore start by detailing the science of gustation, from reception to perception, including its interactions with other sensory systems, before examining its links to genetics and memory, and finally examining supertasting both in humans and other species.
To start with, the gustatory system ‘detects ingested, primarily water-soluble molecules called tastants’ which ‘provide information about the quality, quantity and safety of ingested food’ (Purves et al., 2004, p.337). Once in the mouth, these chemical constituents dissolve in the saliva and interact with receptors located in the tongue, more precisely in epithelial specializations called taste buds – onion-shaped structures containing hundreds taste cells (Purves et al., 2004). These are ‘imbedded under the keratinous layer of the papillae with a taste pore exposed to the external milieu’ (Gravina et al., 2013, p.218). Taste buds are made up of at least five types of cells: glial-like, receptor, presynaptic, basal and neuronal, each playing a role in communicating certain types of information.
Like olfaction, gustation is indeed divided into multiple categories. Classically, they have been limited to five basic qualities: sweet, salty, sour, bitter, and umami. Glial-like (type 1) cells are thus thought to transduce salty taste, presynaptic cells (type 3) detect sour taste along with mediating communication with afferent neurons, while receptor cells (type 2) contain the G-protein coupled receptors (GCPRs) involved in sweet, umami and bitter tastes, and (Gravina et al., 2013). However, evidence now suggests that each taste cell exhibits only one individual modality. ‘For example, a type 2 sweet sensitive cell would express sweet receptors, but would not express bitter or umami receptors and vice versa.’ (Gravina et al., p.218).
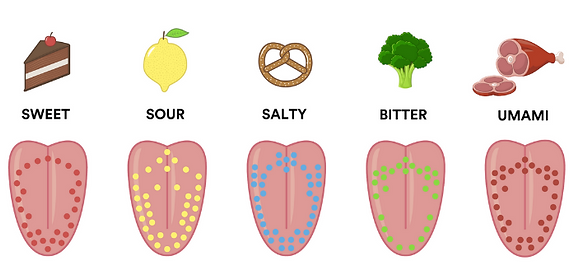
The Five Taste Qualities (alimentarium.org, n.d.)
Moreover, gustation functions in combination with multiple other sensory systems. First of all, the tongue also hosts a majority of filiform papillae that contain trigeminal nerve endings transmitting information on tactile sensations (texture), thermoception (temperature), and nociception (pain). (Gravina et al., 2013). The combination of these three haptic perceptions in the mouth are known as chemesthesis, i.e., the sensitivity of mucosal surfaces to environmental chemicals. Not only does chemesthesis contribute to the overall tasting experience, but it also signals the presence of chemical irritants. Discoveries of such taste receptors outside the mouth has even sparked questions about the nature and scope of the body’s chemoreceptive defence mechanisms (Green, 2011).
Besides, according to Burbidge and Le Révérand (2016), food structure also plays a role in the perception physiology. Indeed, ‘food processes have a tendency to simultaneously create structure, texture and flavour leading to a complex set of interactions that are difficult to separate’ (Burbidge & Le Révérand, 2016, p.3). Structure is defined as the implicit property of a material, as opposed to texture, which is a person’s interpretation of a specific consumption event of a sample. A fine interplay exists between the structural properties of a food and its ability to deliver flavour when interacting with a transport fluid. Unfortunately, direct measurements of their effects on taste perception are not available due to the inaccessible nature of the tongue (Burbidge & Le Révérand, 2016). Lastly, the food molecules released during chewing bind to specific proteinaceous receptors on the tongue as well as in the nasal cavity, which results in a unique pattern of gustatory and olfactory impulses travelling along neurons to the brain (Purves et al., 2004).
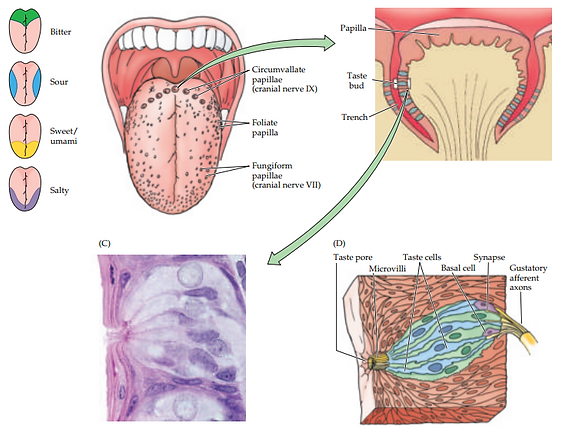
The Gustative System (Purves et al., 2004, p.358)
Lindemann explains that ‘[t]aste transduction typically utilizes two or more pathways in parallel’ (1996, p.719). Much like in other sensory systems, peripheral receptors transduce the stimuli into membrane potential changes that are transmitted to the brain through trains of action potentials. The specificity of gustation lies in the uncertainty regarding the encoding of various aspects of the stimuli, such as quality, intensity and duration in such potentials. Roper (2022) describes this question as ‘hotly debated in the field of taste’ (p.53). On the one hand, information for each different quality is thought to be transmitted along a parallel but separate series of neurons that project to distinct focal clusters of neurons in the gustatory cortex – a concept called labelled line/spatial coding. On the other, taste information could be encrypted by groups of neurons and patterns of impulses within those circuits – a process known as population/combinatorial and temporal coding (Roper, 2022). What is clear, however, is that the brain decodes the combination of information gathered from gustatory, olfactory, trigeminal, and even auditory and visual perceptions to recreate the full picture known as taste (Purves et al., 2004).
In fact, a number of factors influence individual senses of taste. Indeed, variation in taste receptor genes give rise to differential perception of all modalities. For example, studies explain that ‘[o]ver twenty-five bitter taste receptor genes exist, of which TAS2R38 is one of the most studied’ (Feeney et al., 2010, p.135). Inherited variations in this gene cause people to either feel bitter thiourea compounds, such as phenylthiocarbamide (PTC) found in cruciferous vegetables and other foods with purported health benefits, very intensely or not at all – supertasters or nontasters. Several studies even suggest that children who are nontasters of PTC tend to like and consume more dietary fat, making them prone to obesity (Keller & Adise, 2016). Moreover, a 2022 study demonstrated that variation in TAS2R receptor genes explain differential bitterness of two common antibiotics: chloramphenicol and ofloxacin (Nolden et al., 2022). Such research has the potential to optimize delivery systems for improved compliance to medication regimen, especially for oral formulations (Nolden et al., 2022).
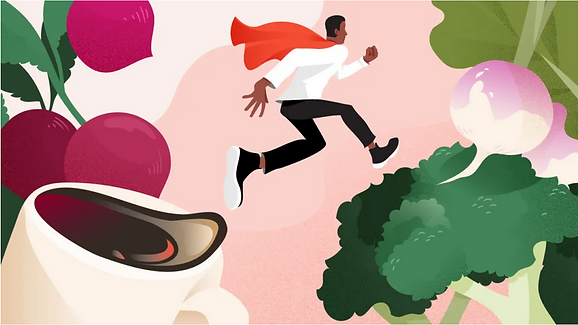
Supertaster (Unknown, 2019)
‘With growing interest in personalized foods, this information is useful for both food product developers and nutrition health professionals alike’, since evidence shows that sensory profiles vary across individuals (Nolden & Feeney, 2020, p.103). It is the case, for instance, of coriander. Dislike of the herb has indeed been linked to the olfactory-receptor OR6A2 gene, a receptor that is highly sensitive to aldehyde chemicals, which contribute to the flavour of coriander. Half of Europeans were found to have two copies of variants that grant the herb a soapy perception, of which 15.3% confirmed that coriander tasted of soap. ‘For comparison, 13% of Europeans had no copies of this variant, and in this group, only 11.5% of them reported the soapy taste’ (Callaway, 2012, p.3). Individual perceptions are therefore thought to be at least partly an inherited trait, so are differences in taster status. However, genetic predispositions to food preferences may be outweighed by environmental or cultural influences (Keller & Adise, 2016).
‘Of course, food is not simply eaten for nutritional value; “taste” also depends on cultural and psychological factors. How else can one explain why so many people enjoy consuming hot peppers or bitter-tasting liquids such as beer?’ (Purves et al., 2004, p.354-5). Furthermore, there is a proven link between taste buds and memory. First, the subregion of the hippocampus responsible for taste memory is strongly correlated with the one encoding the time and place of the experience (Chinnakkaruppan et al., 2014). To continue, a 2018 study suggests that such a correlation originated as a survival tactic called conditioned taste aversion. This ‘learned association between the taste of a particular food and illness such that the food is considered to be the cause of the illness’ was therefore passed down from one generation to the next (Chambers, 2018, p.97).
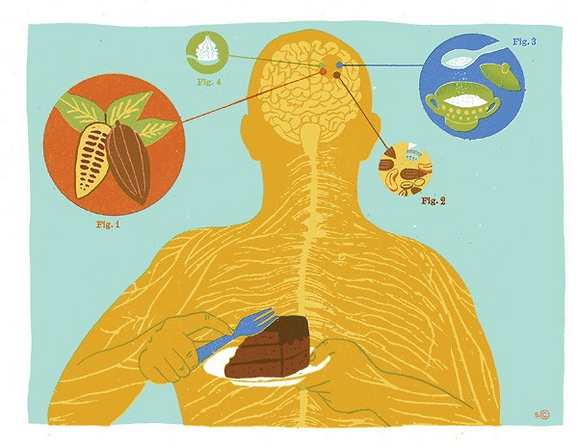
Taste Memory (Casson, 2012)
The primordial importance of gustation for survival is therefore undeniable. The different receptors play a role in evaluating substances to feed on. Sweet taste is indeed believed to have evolved as a way to detect sources of glucose, that is energy, while there is an overall positive correlation between bitterness and toxicity (Beauchamp, 2017). However, while the total number of taste buds in adult humans is estimated at 8,000 (Spence, 2022), many species have developed an even keener gustatory perception. Cows, for example, possess at least 20,000 taste buds, which, as herbivores help them distinguish between poisonous and non-poisonous plants, avoiding bitter-tasting foods while preferring sweet and salty foods, respectively for their high caloric value and electrolyte balance (Marino & Allen, 2017). On the other hand, octopi have a unique nervous system that makes use of chemotactile receptors, meaning they taste their food by touching it. An octopus has around 10,000 taste receptors on each of the 200 suckers on its eight arms. These receptors, combined with their very powerful haptic perception, allow the mollusk to detect incredibly small variations in the concentrations of the water (Van Giesen et al., 2020).
However, the catfish holds the record for best sense of taste, with approximately 100,000 taste receptors. They have a very high sensitivity to amino acids, and unlike for mammals, severing their taste nerve does not eliminate their gustative perception, because their receptors are spread over very large skin areas, often concentrated on their long whiskers or barbels (Atema, 1971). ‘Catfish, described as “swimming tongues”, are unique experimental models for studies of taste reception because of the extensive distribution of taste buds over their external body surface and within their oropharyngeal cavity’ (Caprio et al., 1993, p.192). The visibility in their natural environment being extremely low, the fish rely exclusively on taste to find food. This sense was also found to function as a distance receptor as well as a testing device to control food intake. Such an ability to detect dire quantities of food and locate its exact position demonstrates the vitality of taste for the catfish species (Atema, 1971).
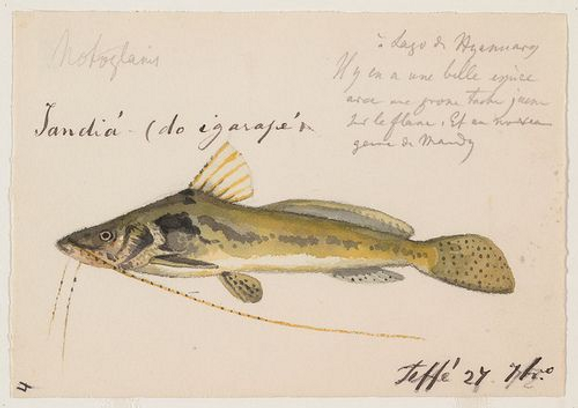
Catfish Scientific Illustration (The Ernst Mayr Library, 1865)
In conclusion, the biophysics of human gustation have been explored over the last decades, bringing to light the various, complex processes that allow the tongue to convey different flavour sensations to the brain. Food quality and intensity can be coded with just five basic modalities, however, the overall experience of taste involves more than merely the tongue: olfaction play a major role, in conjunction with touch, as well as audition and vision. Moreover, while ‘the rich flavors of our human diet are the sum of taste, olfaction, and trigeminal input, but the synthesis of perception is the sum of peripheral input modulated by emotion, physiological and metabolic state, and learning.’ (Gravina et al., 2013, p.222). Taste is indeed intricately linked to memory on top of genetics, while recognition of edible substances is an essential skill that was acquired through trial and error, and passed down from one generation to the next. Future research could focus on the interplay between sensory modality and weight of satiety regulation, or on filiform papillae to better understand the influence on texture on the overall tasting experience, or even on the psychophysics of taste in relation to the entropy of the stimuli, which could provide key new insight into the fields of biology, nutrition, and even medicine.
Bibliographical References
Atema, J. (1971). Structures and functions of the sense of taste in the catfish (Ictalurus natalis). Brain, Behavior and Evolution, 4(4), 273–294. https://doi.org/10.1159/000125438
Beauchamp, G. K. (2016). Why do we like sweet taste: A bitter tale? Physiology & Behavior, 164, 432–437. https://doi.org/10.1016/j.physbeh.2016.05.007
Burbidge, A. S., & Le Révérend, B. J. (2016). Biophysics of food perception. Journal of Physics D: Applied Physics, 49(11), 114001. https://doi.org/10.1088/0022-3727/49/11/114001
Callaway, E. (2012). Soapy taste of coriander linked to genetic variants. Nature. https://doi.org/10.1038/nature.2012.11398
Caprio, J., Brand, J. G., Teeter, J. H., Valentincic, T., Kalinoski, D. L., Kohbara, J., Kumazawa, T., & Wegert, S. (1993). The taste system of the channel catfish: From biophysics to behavior. Trends in Neurosciences, 16(5), 192–197. https://doi.org/10.1016/0166-2236(93)90152-c
Chambers, K. C. (2018). Conditioned taste aversions. World Journal of Otorhinolaryngology – Head and Neck Surgery, 4(1), 92–100. https://doi.org/10.1016/j.wjorl.2018.02.003
Chinnakkaruppan, A., Wintzer, M. E., McHugh, T. J., & Rosenblum, K. (2014). Differential contribution of hippocampal subfields to components of associative taste learning. Journal of Neuroscience, 34(33), 11007–11015. https://doi.org/10.1523/jneurosci.0956-14.2014
Feeney, E., O’Brien, S., Scannell, A., Markey, A., & Gibney, E. R. (2010). Genetic variation in taste perception: Does it have a role in healthy eating? Proceedings of the Nutrition Society, 70(1), 135–143. https://doi.org/10.1017/s0029665110003976
Gravina, S. A., Yep, G. L., & Khan, M. (2013). Human biology of taste. Annals of Saudi Medicine, 33(3), 217–222. https://doi.org/10.5144/0256-4947.2013.217
Green, B. G. (2011). Chemesthesis and the chemical senses as components of a “Chemofensor Complex”. Chemical Senses, 37(3), 201–206. https://doi.org/10.1093/chemse/bjr119
Hara, T. J. (2006). Gustation. Fish Physiology, 45–96. https://doi.org/10.1016/s1546-5098(06)25002-7
Keller, K. L., & Adise, S. (2016). Variation in the ability to taste bitter thiourea compounds: Implications for food acceptance, dietary intake, and obesity risk in children. Annual Review of Nutrition, 36(1), 157–182. https://doi.org/10.1146/annurev-nutr-071715-050916
Lindemann, B. (1996). Taste reception. Physiological Reviews, 76(3), 719–766. https://doi.org/10.1152/physrev.1996.76.3.719
Marino, L., & Allen, K. (2017). The psychology of cows. Animal Behavior and Cognition, 4(4), 474-498. https://dx.doi.org/10.26451/abc.04.04.06.2017
Nolden, A. A., & Feeney, E. L. (2020). Genetic differences in taste receptors: Implications for the Food Industry. Annual Review of Food Science and Technology, 11(1), 183–204. https://doi.org/10.1146/annurev-food-032519-051653
Nolden, A. A., Hayes, J. E., & Feeney, E. L. (2022). Variation in TAS2R receptor genes explains differential bitterness of two common antibiotics. Frontiers in Genetics, 13. https://doi.org/10.3389/fgene.2022.960154
Pallante, L., Malavolta, M., Grasso, G., Korfiati, A., Mavroudi, S., Mavkov, B., Kalogeras, A., Alexakos, C., Martos, V., Amoroso, D., di Benedetto, G., Piga, D., Theofilatos, K., & Deriu, M. A. (2021). On the human taste perception: Molecular-level understanding empowered by computational methods. Trends in Food Science & Technology, 116, 445–459. https://doi.org/10.1016/j.tifs.2021.07.013
Purves, D., Augustine, G., Fitzpatrick, D., Hall, W., LaMantia, A., McNamara, J., & Williams, S. (2004). Neuroscience (3rd ed.). Sinauer Associates, Inc. ISBN 0-87893-725-0.
Roper, S. D. (2021). Encoding taste: From receptors to perception. The Pharmacology of Taste, 53–90. https://doi.org/10.1007/164_2021_559
Spence, C. (2015). Just how much of what we taste derives from the sense of smell? Flavour, 4(1). https://doi.org/10.1186/s13411-015-0040-2
Spence, C. (2022). The tongue map and the spatial modulation of Taste Perception. Current Research in Food Science, 5, 598–610. https://doi.org/10.1016/j.crfs.2022.02.004
Van Giesen, L., Kilian, P. B., Allard, C. A. H., & Bellono, N. W. (2020). Molecular basis of chemotactile sensation in octopus. Cell, 183(3). https://doi.org/10.1016/j.cell.2020.09.008
Zhou, Y., & Tse, C.-S. (2020). The taste of emotion: Metaphoric association between taste words and emotion/emotion-laden words. Frontiers in Psychology, 11. https://doi.org/10.3389/fpsyg.2020.00986
Visual Sources
alimentarium.org. (n.d.). Our Tastes Can Differentiate Between Those Flavours. [Image] https://www.alimentarium.org/en/fact-sheet/senses-taste/
Casson, S. (2012). Taste Memory. [Image] https://www.nature.com/articles/486S7a/
Purves, D., Augustine, G., Fitzpatrick, D., Hall, W., LaMantia, A., McNamara, J., & Williams, S. (2004). Taste buds and the peripheral innervation of the tongue. [Image] Neuroscience (3rd ed.). Sinauer Associates, Inc. 358. ISBN 0-87893-725-0.
Schade, O. (2020). “The Covid 19 Taste” Painting. [Image] https://www.saatchiart.com/art/Painting-The-Covid-19-Taste/1618949/7780709/view/
The Ernst Mayr Library. (1865). Goeldiella eques, Teffe, Brazil. [Image] https://scientificillustration.tumblr.com/post/53738693883/goeldiella-eques-teffe-brazil-27-september/
Unknown. (2019). Supertaster. [Image] https://www.healthline.com/health/food-nutrition/supertaster/
Source: Physics of the Senses 101: Gustatory Perception or Taste